Los sistemas ciber-físicos son una integración sistémica de tecnologías físicas y cibernéticas. Por ejemplo, un automóvil autónomo es una integración de tecnologías físicas, como motores, baterías, actuadores y sensores, y tecnologías cibernéticas, como comunicaciones, computación, inferencia y control. El flujo de datos de las tecnologías físicas a las cibernéticas da como resultado una integración sistémica y la experiencia de conducción deseada. Los sistemas ciber-físicos se están volviendo predominantes en una variedad de sectores, como el militar, la energía, el agua, los residuos, el transporte, la atención médica, la agricultura y la manufactura. A pesar de haber entrado en la era ciber-física, no estamos preparados para este momento debido a la insuficiente asignación sistémica en las ciencias físicas y la falta de planes de estudios de ingeniería multidisciplinarios.
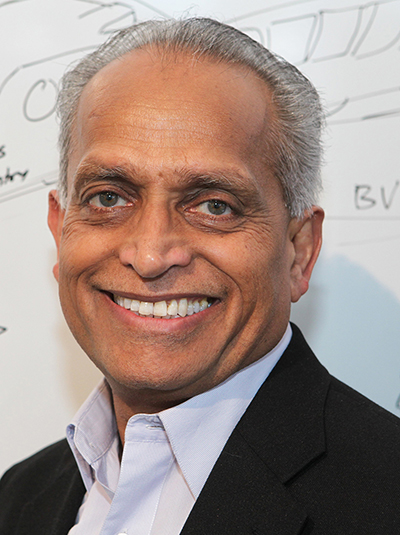
Cyber-physical systems are a systemic integration of physical and cyber technologies. To name one example, a self-driving car is an integration of physical technologies, such as motors, batteries, actuators, and sensors, and cyber technologies, like communication, computation, inference, and closed-loop control. Data flow from physical to cyber technologies results in systemic integration and the desired driving experience. Cyber-physical systems are becoming prevalent in a range of sectors, such as power, water, waste, transportation, healthcare, agriculture, and manufacturing. We have entered the cyber-physical age. However, we stand unprepared for this moment due to systemic under-allocation in the physical sciences and the lack of a truly multidisciplinary engineering curriculum.
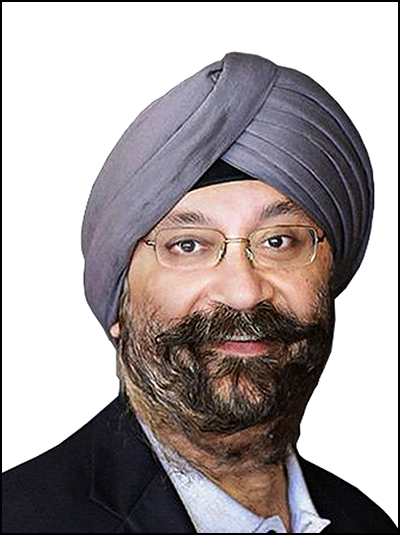
While there are many factors that contribute to the rise of cyber-physical systems, societal challenges stemming from imbalances between supply and demand are becoming a very prominent one. These imbalances are caused by social, economic, and ecological trends that hamper the delivery of basic goods and services. Examples of trends leading to imbalances between supply and demand are resource constraints, aging population, human capital constraints, a lack of subject matter experts in critical fields, physical security risks, supply-chain and supply-side resiliency, and externalities such as pandemics and environmental pollution. With respect to the lack of subject matter experts, consider the supply of cardiothoracic surgeons. The United States has about 4000 cardiothoracic surgeons, a sub-specialization that takes 20 years of education and hands-on training, for a population of 333 million. Similar imbalances in subject matter experts in healthcare, power, water, waste, and transport systems are occurring as a result of aging population. Compounding this challenge is the market-driven pay discrepancy that has attracted our youth to software jobs, such as those in social media, which pay much more relative to the salaries for a resident in general surgery or an early-career civil engineer. While it is possible that the market will shift to value infrastructure- and healthcare-related jobs, the time it takes to train “hands-on” contributors (e.g., engineers and technicians) in physical sciences and life sciences is substantial, ranging from 5 years (technicians requiring industry training) to 20 years (sub-specialized personnel like cardiothoracic surgeons).
One approach to addressing these challenges is to amplify human capacity with cyber-physical systems. For example, virtual reality (VR) systems with 3D immersive visualization and haptic interfaces can be used by one subject matter expert to remotely manage and conduct many complex tasks. These VR systems can also include pupillometry, vital signs measurements, and other techniques to discern the cognitive load on the subject matter expert managing the complex tasks and ensure an adequate level of safety. Similarly, VR systems can also be used by one subject matter expert to train many. Robotics and automation are other examples of cyber-physical systems that can offer solutions to supply-and-demand-related challenges. In the case of surgeons, both general and sub-specialized (e.g., cardiothoracic surgeons), robotic surgery can help mitigate the imbalance between the supply of surgeons and the demand for them. Robotic surgery systems with immersive human-machine interfaces, precision actuators, sensing, and control can enable expeditious and exacting operations. Surgeons, given their deep domain knowledge from decades of training, can also play a key role in the burgeoning field of artificial intelligence (AI). They can devise AI automation tools to expedite pre-surgery, surgery, and post-surgery outcomes.
Cyber-physical systems can also rise to the supply-and-demand-related challenges associated with ecological problems and resource constraints. The scarcity of drinking water due to the vagaries of climate change and the energy required to treat and distribute drinking water pose a formidable challenge. Diurnal treatment and distribution of drinking water to a region of 3 million people, such as Silicon Valley, requires the allocation of a large 1 GW power plant for one hour. Furthermore, in some geographies, energy must be allocated to desalinate and extract drinking water. The objective of the cyber-physical management of drinking water is to minimize the energy required for extraction, distribution, use, upcycling, and recycling. Managing supply and demand by integrating gates, pumps, valves, sensors, communications, computation, inference, and control is a way to minimize the lifetime energy of water. Holistic cyber-physical security that transcends securing cyber and data components to include physical systems and operations is an additional salient element of the overall system.
While cyber-physical systems hold immense promise, systemic under-allocation in the physical sciences — in areas such as research funding, venture capital, new business formation, patents issued, capital-intensive physical science education, and the development of human resources — has left the United States ill-prepared for the cyber-physical age. During 2021, 40% of venture capital funding went to software start-ups, about three times higher than the next big area in biotech and pharmaceuticals [1]. From 2010 to 2020, SaaS (software as a service) funding went up sevenfold. In 2021, 27 SaaS companies went public, representing $227 billion of market capital [2]. Meanwhile, the government funding for research in the physical sciences during this period remained relatively flat [3].
In our own decades-long experience teaching college classes and mentoring students and practitioners in Silicon Valley, from the days of local design and manufacturing of hardware and software to the present, we have observed an erosion of hands-on training in the physical sciences. In particular, we have noticed a decline in the hands-on training of technicians in community colleges and regional occupation programs and “learn-by-doing” apprentice training in hardware design and manufacturing in the industry. The result of these trends is a dearth of people who are trained to work on systems that integrate physical and cyber technologies.
Despite these concerning trends, there are now ambitious targets related to cyber-physical systems at the policy level for sustainability and shifting design and manufacturing from abroad back to the United States. The 2022 US CHIPS and Science Act aims to boost domestic research and manufacturing of semiconductors. That act, which plans to provide approximately $280 billion in new funding, will require legions of cyber-physical practitioners. Substantive outcomes in environmental sustainability will likewise require practitioners grounded in physical domain fundamentals, data, and data science.
In turning the tide to achieve the scale cyber-physical systems require, we must begin with education to ignite a passion for cyber-physical systems at home, on social media, in our schools, and on our college campuses. Just as cyber-physical systems integrate physical and cyber technologies, the cyber-physical practitioner requires a curriculum that results in depth in physical science and breadth in cyber science and other subjects. We ought to define job types in research and development that require a BS and an MS in a subject related to physical science (e.g., mechanical engineering, materials science, chemical engineering, bioengineering) and a PhD in cyber science. Likewise, there should be other job types that require a BS in physical science-related subjects and an MS in cyber science. Additional courses — in economics, other social sciences, and the humanities — required for the respective degrees must remain. Indeed, the objective of sustainability necessitates operating at the intersection of social, economic, and ecological spheres that transcend engineering subjects.
It is also imperative that we shape the curriculum in each degree program — the courses in the physical sciences and the cyber sciences — to take advantage of advances such as generative AI. Consider a mechanical engineer analyzing rotating machinery such as a turbine. Presented with the need to convert time-varying physical signals and analyze them with respect to frequency, the engineer can simply pose a question that presents the Fourier transform code needed to perform the time-to-frequency conversion. Generative AI expedites the creation of the syntax of the software code that, before, required training in programming and looking up detailed documentation. Such democratization in software creation provides a unique opportunity to reshape the curriculum and expedite the training of cyber-physical practitioners.
The well-prepared cyber-physical practitioner of tomorrow should have a holistic perspective that includes social, economic, ecological, and technical know-how. A simple measure of success in the year 2030 will be the answer to a question posed to a Silicon Valley practitioner: What is the source of your drinking water? It is a question that provides a lesson in the difficult choices that must be made in balancing sustainability with the burgeoning demand-side needs of the region. We will expect a holistic answer that starts at the top water level and the gates of a source such as the Hetch Hetchy Reservoir formed by O’Shaughnessy Dam in the Sierra Nevada mountains. We will expect an understanding of the ecological and social challenges debated more than 100 years ago by Chief Engineer Michael O’Shaughnessy, who wanted to construct a dam that would provide pristine water to the San Francisco Bay Area, and John Muir, who wanted to preserve the valley that was as beautiful as Yosemite. We will further expect the Silicon Valley practitioner to grasp the need to build cyber-physical systems capable of managing, judiciously utilizing, and preserving the critical physical infrastructure. The goal of sustainability requires us to preserve the critical physical infrastructure so that current and future generations can enjoy the same quality of life we have enjoyed, or hopefully an even better one.
Acknowledgment
We deeply appreciate the guidance and feedback from Editor Kyle Gipson and the evaluators in shaping this piece. We thank them.
Notes